The high-frequency switching of WBGs presents challenges related to bandwidth and speed that can be addressed with new sensing techniques. In addition, gallium nitride (GaN) and silicon carbide (SiC) devices have different tolerances to short circuit conditions and requirements for current sensing.
Current sensors with the bandwidth needed to capture ultra-fast short-circuit events can be critical when using GaN devices, which have a much lower short circuit withstand time than Si and SiC devices. As a result, current sensors used in Si-based power converters are often also suitable for use in SiC applications but not with GaN.
For example, Rogowski coils (RCs) are common in high-power applications since they can provide galvanic isolation, make high current measurements, and are simple to integrate. However, RCs’ bandwidth is limited to tens of MHz, and as discussed below, efforts are being made to design a new generation of RCs with bandwidths of 3 GHz and higher for use with WBGs.
The limited bandwidths of many existing current sensors make using them with SiC and GaN devices difficult. The bandwidth requirements of SiC devices (BWSiC) exceed 100 MHz, and for GaN devices, BWGaN exceeds 500 MHz (Figure 1). The limited bandwidths of many existing sensing technologies make them challenging or unsuitable for WBG use. As a result, new current sensing techniques are being explored and developed.
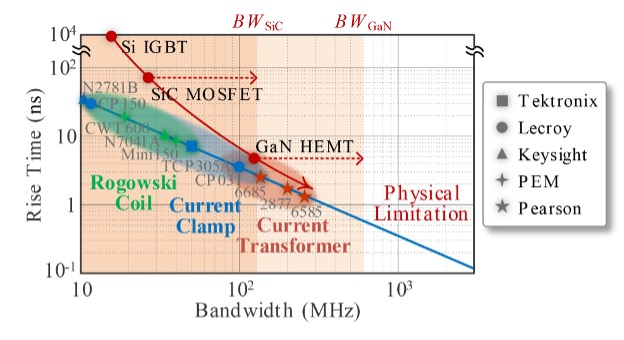
This discussion focuses on the bandwidth requirements of WBG applications. Still, factors like isolation capability, size, ability to measure DC currents, EMI immunity, thermal stability, accuracy, linearity, power consumption, and cost vary widely. Isolation can be essential for high-voltage applications, and EMI immunity is often an important consideration when using high-frequency and high-wattage WBG-based power converters.
Most current sensing technologies struggle to reach tens of kHz bandwidths, and even high-bandwidth devices are generally limited to tens of MHz, which is not enough for many WBG applications (Figure 2).
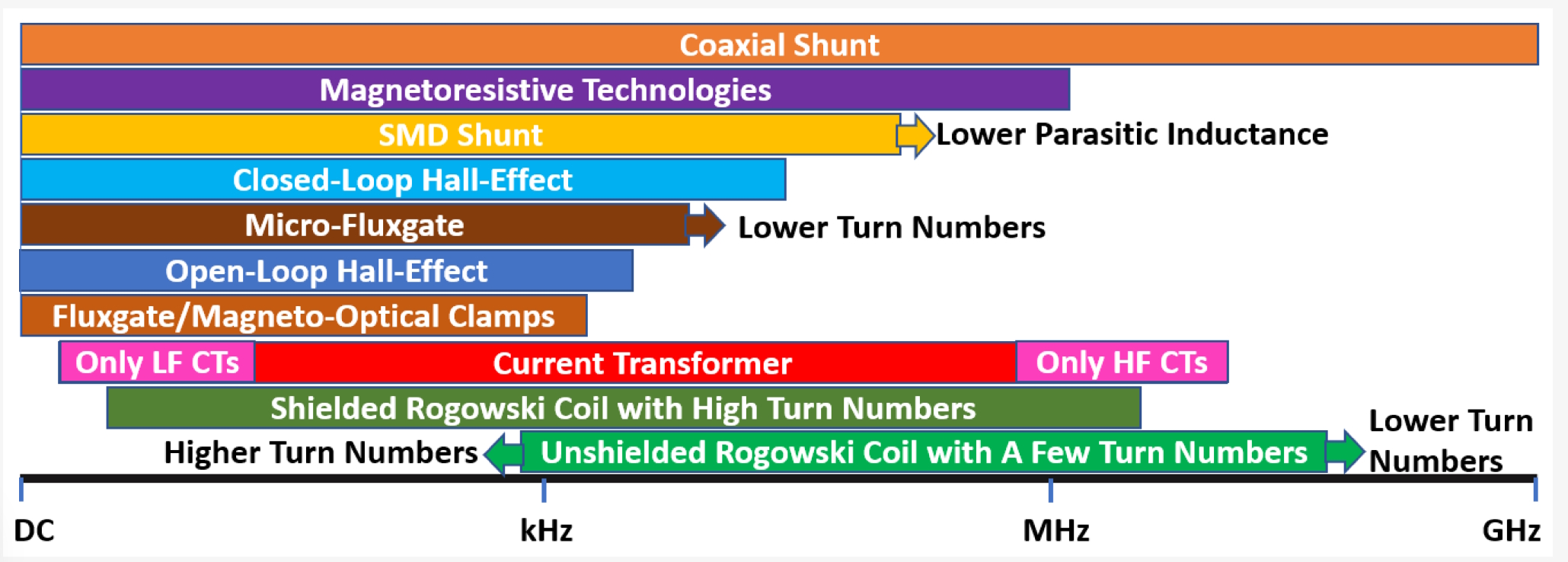
Improved Rogowski coil
For high-power WBG applications, an improved RC has been developed that expands the bandwidth from tens of MHz to 3+ GHz. In a conventional RC, the bandwidth is limited by the resonances that occur due to self-inductance and parasitic capacitance interactions. The new transmission line (TL) RC addresses the limitations of inductance and capacitance. The sensor also employs double-ended impedance matching to ensure high signal integrity at GHz levels.
Device-integrated current sensing
Different integrated current sensing approaches have been developed for enhancement-mode, e-mode, (usually off) GaN devices, and cascode devices combining a normally-on GaN HEMT and a normally-off Si MOSFET.
A cascaded GaN half-bridge with a 17 MHz bandwidth galvanically isolated current sensor has been developed. When integrated into a half-bridge module, the new IC improves switching frequency and reduces the size of capacitors and inductors, contributing to a smaller solution size. It consists of a 650 V depletion-mode GaN FET switched by a low-propagation-delay gate driver using a 25 V N-channel laterally diffused MOS FET (LDMOS).
The LDMOS also serves as a shunt resistor for current sensing. Process, voltage, and temperature (PVT) variations of on-resistance of the current-detecting LDMOS are compensated using a reference LDMOS. A digital calibration loop across the isolation barrier keeps the current sensor gain constant within ±1.5% over the operating temperature range (Figure 3)
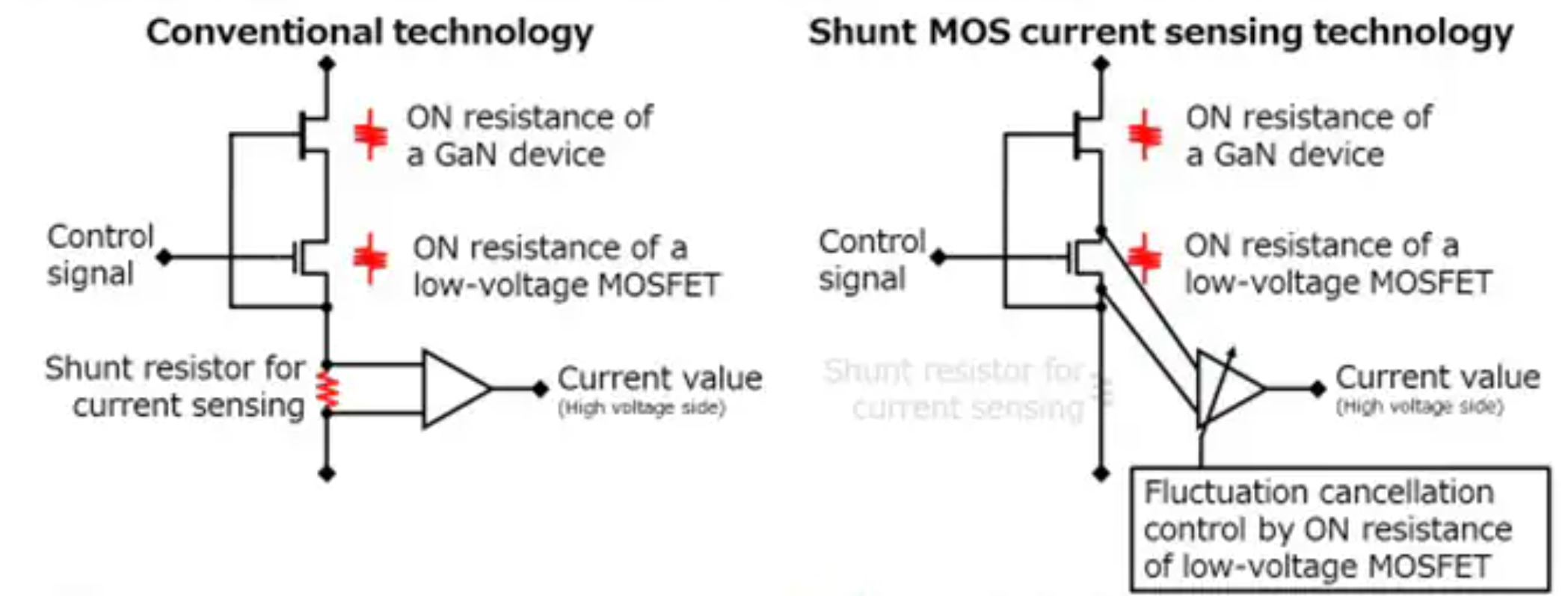
In another example, a small “current mirror” has been fabricated monolithically in parallel with an e-mode GaN power FET. The resistance of the sensing “mirror” FET is up to 1,500 larger than the main power FET, resulting in negligible power loss from current sensing. In addition, being monolithically integrated, the on-resistance and temperature effects of the two devices cancel and have no impact on current sensing accuracy. The over-current sensing time can be as little as 30 ns, compared with 180 ns for traditional approaches (Figure 4).
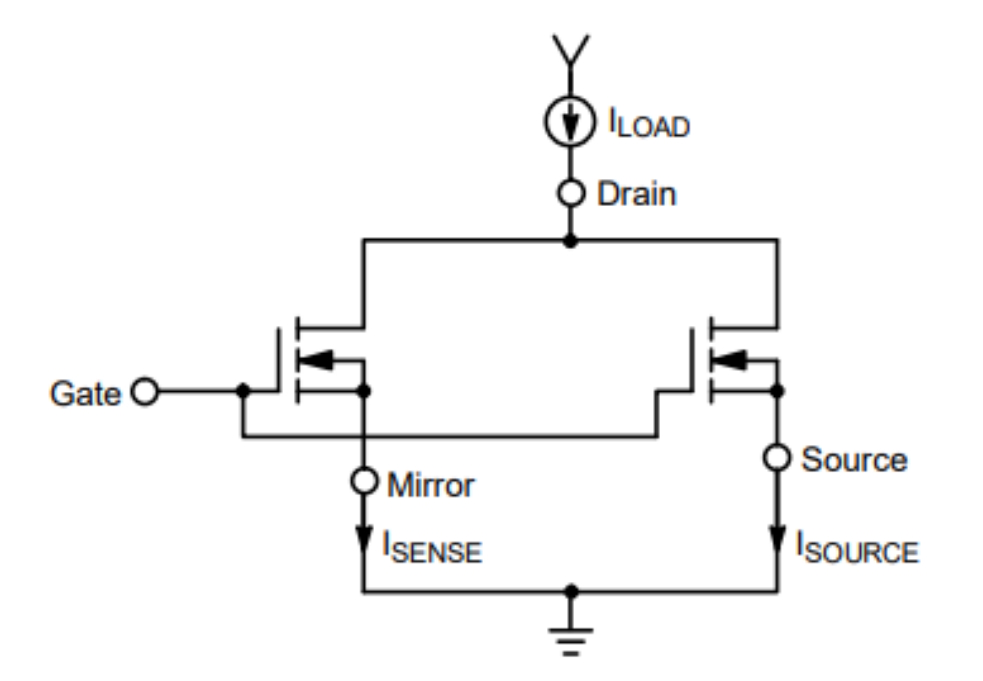
Summary
Numerous challenges related to current sensing when using WBGs exist. Bandwidth and speed are among the most important ones. Most existing current sensing technologies are not suited for use with WBGs. As a result, several groups are working on alternatives, ranging from improved RCs to various ways of integrating current sensing with WBG devices.
References
Cascaded GaN half-bridge with 17 MHz wide-band galvanically isolated current sensor, Japanese Journal of Applied Physics
Current Sensor Integration Issues with Wide-Bandgap Power Converters, MDPI sensors
The Past, Present, and Future of Current Sensing, Navitas Semiconductor
Transmission Line Rogowski Coil: Isolated Current Sensor with Bandwidth Exceeding 3 GHz for Wide-Bandgap Device, IEEE Transactions on Power Electronics
Leave a Reply