Power-generation and heating units using radioactive decay as their primary energy source have been successfully used in space and on Earth for over 60 years.
This part looks at RTG operation in detail.
Q: What’s this about radioactive decay and heat?
A: Heat is generated when the natural or man-made unstable isotopes of elements undergo radioactive decay. Scientists believe that at least half the heat of the Earth comes from such decay in Earth’s crust and mantle, while the rest of the heat is primordial remains from the formation of the Earth. Uranium, thorium, and potassium are the main elements contributing to natural terrestrial radioactivity. Their isotopes 228U, 235U, 232Th, and 40K decay with half-lives so long that significant amounts remain in the Earth.
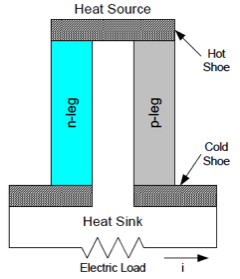
Q: What is in an RPG or an RTU?
A: An RTG is conceptually simple. It uses the natural radioactive decay of an isotope of plutonium, 238Pu, (also spelled out as plutonium-238) as the heat source. Thermocouples capture this heat and convert it into electricity using the well-known Seebeck principle, where two connected dissimilar metals create a potential difference and thus a current flow across their junction (Figure 1).
The natural decay of Pu-238 provides the heat required to raise the temperature of the hot side of the thermocouple, and the ambient environment lowers the temperature of the cold side of the thermocouple, via the generator housing. Since each individual thermocouple can provide only a small voltage difference, many thermocouples connected in series provide the required system output voltage.
Q: Why plutonium?
A: There are multiple factors that must be considered.
For a limited-volume source, such as in an RTG, the generation of heat takes place across a relatively short length within the device walls. This favors decay products that have short absorption lengths. For the three types of radioactive decay (alpha, beta, gamma), the order of radiation absorption lengths is alpha, beta, and then gamma going from shortest to longest. Therefore, for the material contained within an RTG, most heat will be produced by alpha decay, so it is best to find isotopes that decay with alpha radiation first.
The other consideration is decay half-life; the length of time it takes for the radioactive output to drop to half its original value. Obviously, you want a half-life that is commensurate with the mission length.
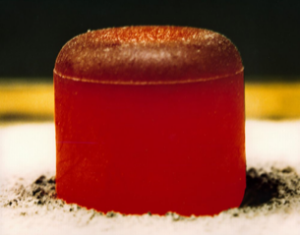
Q: Where does this analysis lead?
A: The most frequently used isotopes for RTG fuels include Plutonium-238 (Pu-238), Strontium-90 (Sr-90), and Curium-244 (Cm-244), with Pu-238 being the most common choice. Pu-238 satisfies all the above RTG fuel requirements, offering high radiation output that is primarily alpha decay, and thus has low shielding needs, a very long half-life of just under 88 years, and a fuel pellet packaged into the size of a marshmallow, fabricated in the form of plutonium dioxide (Figure 2).
Other isotopes can also serve as fuels but have various disadvantages in comparison with Pu-238, including the need for shielding due to non-alpha radiation decay, shorter half-lives, and generally less radiation output.
Q: Where does this plutonium come from?
A: Due to its natural decay, plutonium is very rare on Earth, and is only found in minuscule trace amounts in uranium. In reality, all forms of plutonium — the basic element and isotopes — are created from uranium in nuclear reactors by irradiation of natural uranium with neutrons followed by beta decay. It is a by-product of nuclear weapons production and nuclear power operations. Availability of Pu-238 has been a problem, as some of the reactors which produce it were shut down for various reasons. Additionally, you can’t make more than you need and just store the excess, as it inherently and unavoidably decays while “on the shelf.”
Q: What’s the operating decay rate for an RTG?
A: The two decay factors are the half-life of Pu-238 and the degradation of the thermocouples due to various subtle but understood factors. The output power degradation due to radioactive decay of the Pu-238 causes results in degradation at ~0.8% per year. Thermocouple degradation is ~0.8% per year, depending on the material and the operating conditions.
Q: Is the Seebeck effect used only for scientific applications?
A: Absolutely not. It is widely used in standard thermocouples as a sensor — not a power source — to measure temperature accurately over a wide range, and it is also used in some consumer appliances. For example, older gas-powered water heaters used the heat of the pilot light (they are no longer permitted due to energy-saving mandates) under an array of thermocouples to generate a small voltage. This voltage powered a gas-flow safety valve to keep it open. If the pilot light went out for any reason, the thermocouple output dropped to zero and the valve would close. This prevented the flow of raw gas to the pilot light assembly and tank’s burner which would not be combusted but would fill the area with gas and present a major explosion hazard.
Q: What are the RTG or RTU thermocouples made of?
A: Thermocouples used in RTGs and RTUs contain high-performance thermoelectric materials such as bismuth telluride (BiTe), lead telluride (PbTe), tellurides containing antimony, germanium, and silver (TAGS), and silicon germanium (SiGe). These materials absorb the heat generated by the RTG isotope fuel, create a dramatic temperature gradient due to their low thermal conductivities, and then produce electrical currents that are outputted by the RTG to elements that need to be powered.
Part 4 continues to look at RTG implementation.
Related EE World Content
Thermal electric generators, Part 1: Principles and implementation
Thermal Electric Generators, Part 2: Applications
Gravity-assist “Slingshot,” Part 1: Background and principle
Gravity-assist “Slingshot,” Part 2: Application
Making sense of thermocouples and interfaces (Part 1)
External References (with some comments)
NASA, JPL, Dept. of Energy
- NASA/JPL, “Radioisotope power systems reference book for mission designers and planners” (Detailed, fact-packed 91-page book!)
- NASA/JPL. “A Look at the Next Generation of Radioisotope Thermoelectric Generators” (15 slides, excellent)
- NASA, “Cassini Radioisotope Thermoelectric Generators (RTGs)”
- NASA, “After 60 Years, Nuclear Power for Spaceflight is Still Tried and True”
- NASA, “RHU Pull-Apart Animation”
- NASA, “FAQ About RPS” (very useful)
- NASA, “A Step Forward in Reestablishing the Radioisotope Power Systems Supply Chain” (plutonium production issues)
- NASA, “Power Systems” (summary with video of RTGs)
- NASA, “Thermal Systems” (summary with video of RTUs)
- NASA, “NASA’s RHU-Heated and RTG-Powered Spacecraft” (broad overview)
- NASA, “So What’s an RTG and Are They Safe?
- NASA, “General Purpose Heat Source – Light-Weight Radioisotope Heater Unit”
- NASA, “RHU Fact Sheet”
- S. Department of Energy, “What is a Radioisotope Power System?”
- S. Department of Energy, “The History of Nuclear Power in Space”
- S. Department of Energy, “Overview of Light Weight Radioisotope Heater Unit (LWRHU) User’s Guide”
Other sources
- American Nuclear Society, Science and Technology Policy Institute,” Current Status and Future of Space Nuclear Power” (provides various technical and policy perspectives)
- Stanford University, “An Overview of Radioisotope Thermoelectric Generators”
- Wikipedia, “Radioisotope heater unit”
- Wikipedia, “Radioisotope thermoelectric generator”
Earth’s heat and radioactivity
- University of Notre Dame Nuclear Science Laboratory “Radioactivity, Lecture 11: The radioactive Earth”
- Physics World, “Radioactive decay accounts for half of Earth’s heat”
- Scientific American, “Do transuranic elements such as plutonium ever occur naturally?”
- Lawrence Berkeley National Laboratory “What Keeps the Earth Cooking?”
Leave a Reply